Research topics
Acoustic Simulation
Nonlinear Wave Equation and Its Application such as Parametric Array
Model
Equation
Range
Limitation
Kuznetsov equation
\( \nabla^2 p – \frac{1}{c_0^2}\frac{\partial^2 p}{\partial t^2} \color{red}{+ \frac{\delta}{c_0^4}\frac{\partial^3 p}{\partial t^3}} \color{blue}{+ \frac{\beta}{\rho_0 c_0^4}\frac{\partial^2 p^2}{\partial t^2}} \color{white}{+ \left(\nabla^2 + \frac{1}{c_0^2} \frac{\partial^2 }{\partial t^2} \right) L} = 0\)
Propagation, Reflection, near-field (3D model)
High computational cost
Westervelt equation
Propagation, Reflection (3D model)
Invalid in near-field
KZK equation
Propagation, Sound beam (2D Axisymmetric model)
Valid within 20˚ from the main axis
Burgers’ equation
Plane-wave (1D model)
Neglect the diffraction effect
There are various nonlinear acoustic equations, and each equation contains advantages and disadvantages. The Kuznetsov equation can be accurately calculated up to the near field, but has the disadvantage of requiring a long calculation time. Burgers’ equation is only valid in one dimension, so it does not take into account the diffraction effect. Therefore, the Westervelt equation and the KZK equation have been mainly used for the design of a parametric array. Black, red, and blue represent the linear wave equation, absorption, and nonlinearity term, respectively.
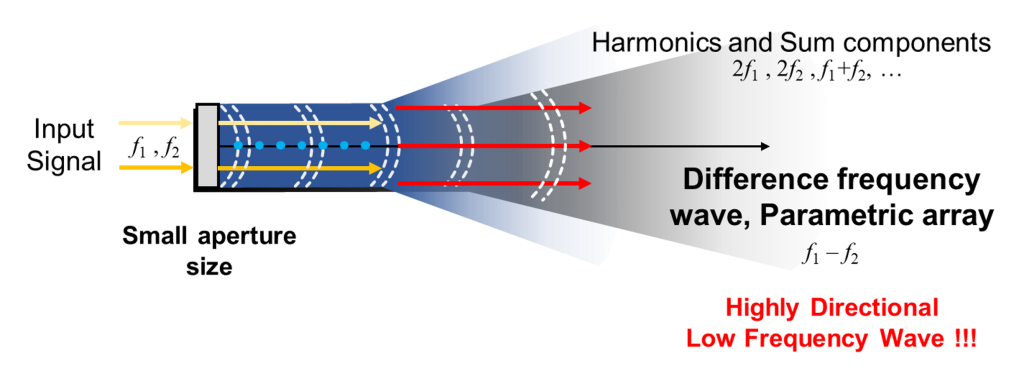
The parametric array phenomenon occurs due to the nonlinear phenomenon of the medium. When the transducer strongly generates two frequencies f1 and f2 with highly directive sound waves, nonlinear distortion gradually occurs as these sound waves travel, creating a virtual line array source (blue dots) as shown in the figure. When a secondary wave with such a high directivity is generated, the high-frequency components disappear rapidly due to absorption, and only the low-frequency components corresponding to the difference frequency remain. Through this parametric array phenomenon, it is possible to obtain high-directivity low-frequency sound.
Analysis of Beam Steering of Difference Frequency by Parametric Array
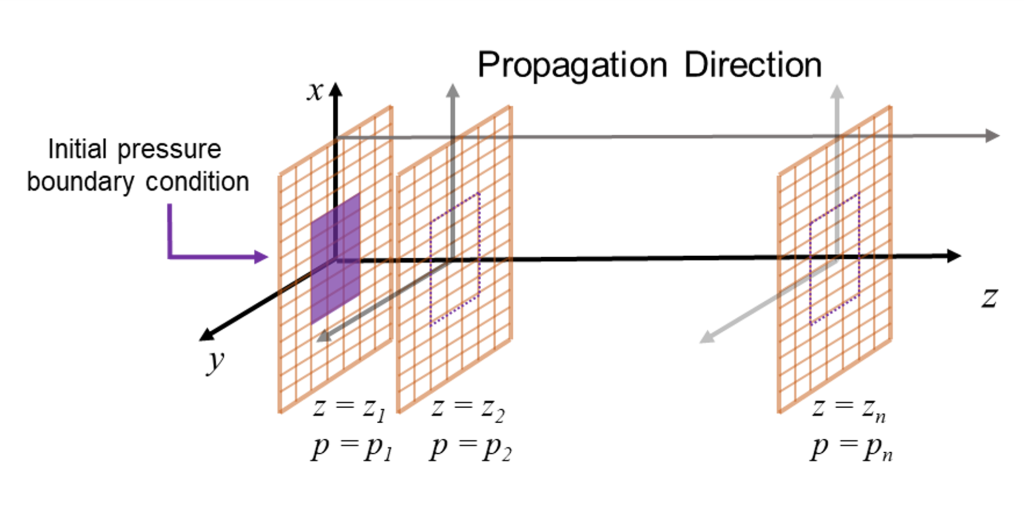
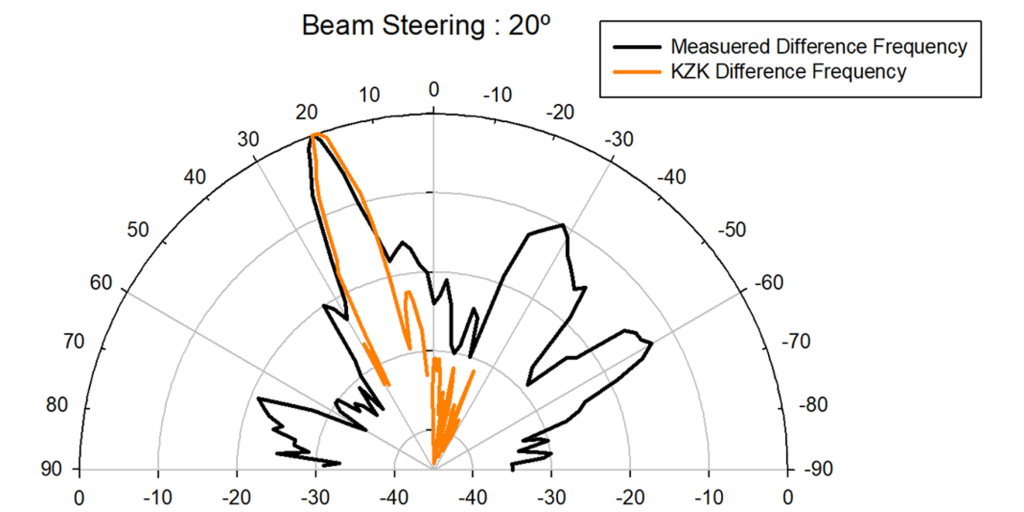
Our laboratory calculates the beam pattern of difference frequency using the KZK equation, and verifies the simulation results by comparing them with the experimental results. When the difference frequency wave formed along the main axis, very similar results are obtained from the experimental results and the results of the KZK equation. The difference frequency is formed by setting the steering angle to 20 degrees. The phased array technique can change the direction of audible sound without directive mechanical movement by applying an appropriate time delay to each unit.
Related Publications
- Been, K., W.-S. Ohm, and W. Moon, Computational study on prediction of electrical beam steering phenomenon of parametric array sound source. The Journal of the Acoustical Society of Korea, 2019. 38(5): p. 485-493.
- Been, K. and W. Moon, Reconsideration on Helmholtz-Kirchhoff Integral Solutions for Boundary Points in Radiation Problems. Acta Acustica United with Acustica, 2019. 105(5): p. 827-837.
- Been, K., S. Nam, H. Lee, H.S. Seo, and W. Moon, A lumped parameter model of the single free-flooded ring transducer. Journal of the Acoustical Society of America, 2017. 141(6): p. 4740.
Parametric Array Loudspeaker
We are developing a directional speaker system based on parametric array technology to achieve precise and controlled sound distribution. Unlike conventional speakers that disperse sound in a broad area, our system uses ultrasonic carrier waves modulated with an audio signal to generate highly-directional sound beams through nonlinear interaction in the air. This is ideal for applications where multiple audio streams must coexist without interference, such as museums, conventions, and private listening spaces. Our prototype system delivers clear and focused audio over several meters, with adjustable beam angles and an audio frequency range spanning the human audible spectrum.
Stepped Plate Parametric Array Loudspeaker
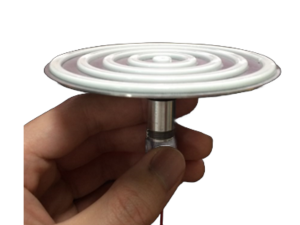
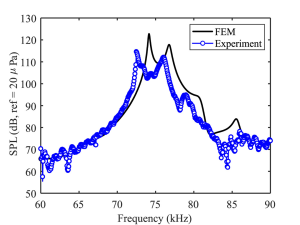
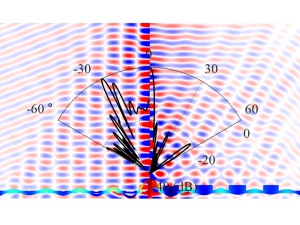
In our laboratory, we are pioneering research on the integration of stepped plates with parametric array technology, a highly innovative approach in the field of acoustics. The parametric array is a nonlinear acoustic technology that generates a narrow difference frequency sound beam by utilizing the interaction between two high-frequency waves at the small radiating surface. Since most of the parametric array loudspeakers consist of multiple actuators such as an array structure, the parametric array loudspeaker is expensive, preventing popularization. By incorporating stepped plates with an ultrasonic transducer, we devise a novel type of parametric array loudspeaker with a single actuator, making it cost-effective.
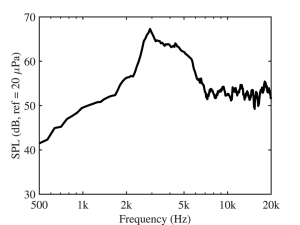
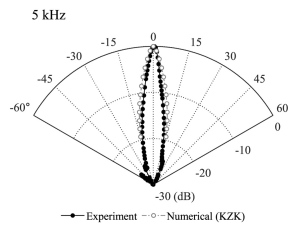
Related Publications
- Oh, B., C. Kim, D. Lee, J. Rho, and W. Moon, An improved analytic model for designing the polymer-composite stepped-plate transducer using the modified Mindlin plate theory. Ultrasonics, 2023. 131: p. 106933.
- Kim, C., Y. Hwang, K. Shin, and W. Moon, A parametric array loudspeaker featuring a Langevin transducer, a circular aluminum plate, and composite polymer steps. Sensors and Actuators a-Physical, 2022. 338: p. 113466.
- Hwang, Y., W. Moon, Y. Je, W. Kim, and S. Lee, A Precise Equivalent-Circuit Model for a Circular Plate Radiator. Acta Acustica United with Acustica, 2018. 104(1): p. 79-86.
- Hwang, Y. and W. Moon, A wide-band in-air parametric array source composed of a circular plate with steps and a Langevin transducer with a horn. Applied Acoustics, 2018. 141: p. 223-233.
- Je, Y., H. Lee, J. Park, and W. Moon, A stepped-plate bi-frequency source for generating a difference frequency sound with a parametric array. Journal of the Acoustical Society of America, 2010. 127(6): p. 3494-502.
pMUT Parametric Array Loudspeaker
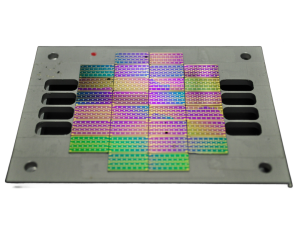
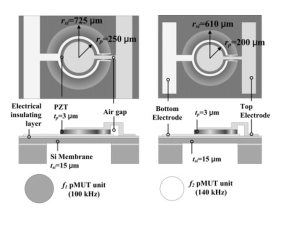
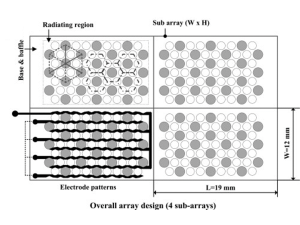
In our laboratory, we focus on the development and characterization of Piezoelectric Micromachined Ultrasonic Transducers (pMUTs), a state-of-the-art technology in the field of ultrasonics. pMUTs, a category of micro-electromechanical systems (MEMS), are known for their capabilities to generate and receive ultrasonic waves, making them a key component in a variety of applications, including medical imaging, industrial non-destructive testing, and acoustic sensing.
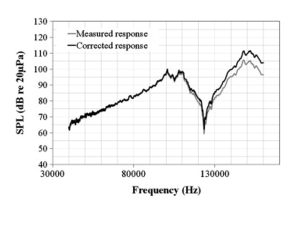
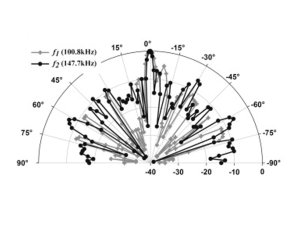
Related Publications
- Ahn, H.M., Y. Hwang, Y. Je, and W. Moon, Practical method for parametric array source evaluation at 120 kHz frequency range in the near-field region. Noise Control Engineering Journal, 2020. 68(5): p. 389-398.
- Ahn, H., K. Been, I.D. Kim, C.H. Lee, and W. Moon, A Critical Step to Using a Parametric Array Loudspeaker in Mobile Devices. Sensors (Basel), 2019. 19(20).
- Je, Y., H. Lee, K. Been, and W. Moon, A micromachined efficient parametric array loudspeaker with a wide radiation frequency band. Journal of the Acoustical Society of America, 2015. 137(4): p. 1732-43.
- Je, Y., H. Lee, and W. Moon, The impact of micromachined ultrasonic radiators on the efficiency of transducers in air. Ultrasonics, 2013. 53(6): p. 1124-34.
- Lee, H., D. Kang, and W. Moon, A micro-machined source transducer for a parametric array in air. Journal of the Acoustical Society of America, 2009. 125(4): p. 1879-93.
Polymer-based MEMS Microphone
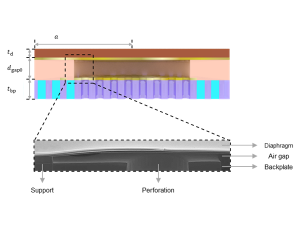
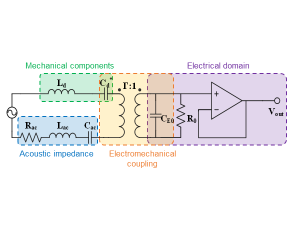
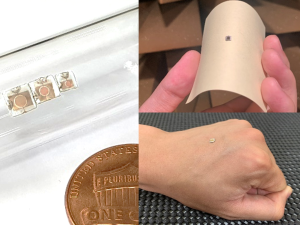
We developed a highly advanced skin-attachable acoustic sensor designed for wearable applications in sound recognition systems for enhanced human-machine interaction and IoT devices. Unlike previous wearable sensors with limited sound-sensing capabilities, this sensor offers exceptional performance. It boasts a flat frequency response covering the range of 15-10,000 Hz, a wide linear acoustic pressure range of 29-134 dB SPL, and remarkable flexibility for conforming to various surfaces, including human skin.
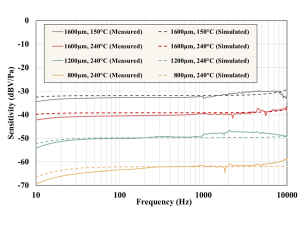
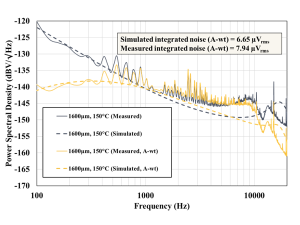
Related Publications
- Lee, S., H. Roh, J. Kim, S. Chung, D. Seo, W. Moon, and K. Cho, An Electret‐Powered Skin‐Attachable Auditory Sensor that Functions in Harsh Acoustic Environments. Advanced Materials, 2022. 34(40): p. 2205537.
- Lee, S., J. Kim, H. Roh, W. Kim, S. Chung, W. Moon, and K. Cho, A High-Fidelity Skin-Attachable Acoustic Sensor for Realizing Auditory Electronic Skin. Advanced Materials, 2022. 34(21): p. e2109545.
- Kim, J., S. Lee, G.Y. Bae, and W. Moon, Evaluation of Polymer Materials for MEMS Microphones. Journal of Microelectromechanical Systems, 2022. 31(4): p. 673-682.
- Lee, S., J. Kim, I. Yun, G.Y. Bae, D. Kim, S. Park, I.M. Yi, W. Moon, Y. Chung, and K. Cho, An ultrathin conformable vibration-responsive electronic skin for quantitative vocal recognition. Nature Communications, 2019. 10(1): p. 2468.
Electret Gate on a Field-Effect Transistor Microphone
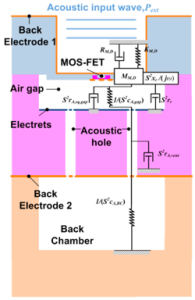
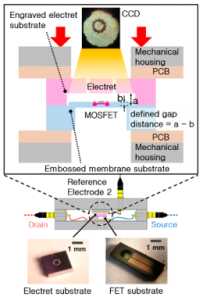
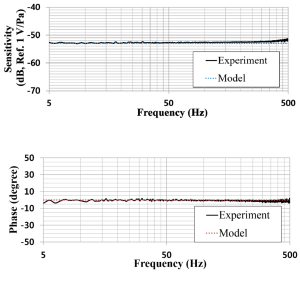
Conventional capacitive microphones have sensitivity issues in miniaturized, particularly in detecting low-frequency sounds due to electrical and acoustical time constants. capacitive microphones involve large diaphragms and chambers to extend time constants. To overcome these limitations, we developed the Electret Gate on Field-Effect Transistor (ElGoFET) structure, where a diaphragm-mounted field-effect transistor (FET) interfaces with an electret. This innovative integration of FET and electret allows direct diaphragm displacement detection, leading to the development of the ElGoFET microphone with strong low-frequency sound detection capabilities. Our microphone demonstrates feasibility as a low-frequency Micro-Electro-Mechanical System (MEMS) microphone, offering advantages for miniaturized component applications without high DC bias voltage.
Related Publications
- Shin, K., C. Kim, M. Sung, J. Kim, and W. Moon, A Modeling and Feasibility Study of a Micro-Machined Microphone Based on a Field-Effect Transistor and an Electret for a Low-Frequency Microphone. Sensors (Basel), 2020. 20(19): p. 5554.
- Shin, K., J. Jeon, J.E. West, and W. Moon, A Micro-Machined Microphone Based on a Combination of Electret and Field-Effect Transistor. Sensors (Basel), 2015. 15(8): p. 20232-49.
Piezoelectric Gate on a Field-Effect Transistor Hydrophone
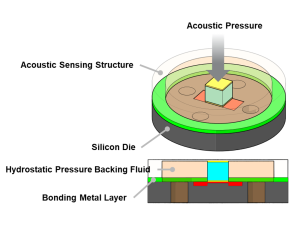
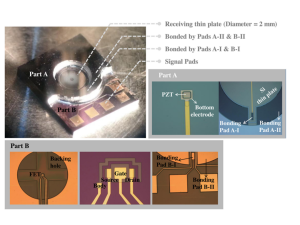
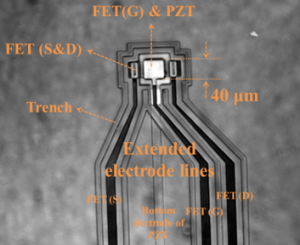
We reported a micro-machined hydrophone using piezoelectric gate on a field-effect transistor (PiGoFET). This novel transduction mechanism decouples sensitivity from piezoelectric body dimensions, allowing hydrophone miniaturization. We achieved this via hybrid bonding integration, creating a micro-PiGoFET in a CMOS-compatible process. Our micro-PiGoFET hydrophone exhibits a sensitivity of -191.5 dB ± 1 dB (Ref. 1 μV/Pa) for frequencies spanning 50–1000 Hz. These results highlight the potential for high-performance miniaturized hydrophone systems suitable for wide-band and low-frequency applications, including system-on-chip functionality.
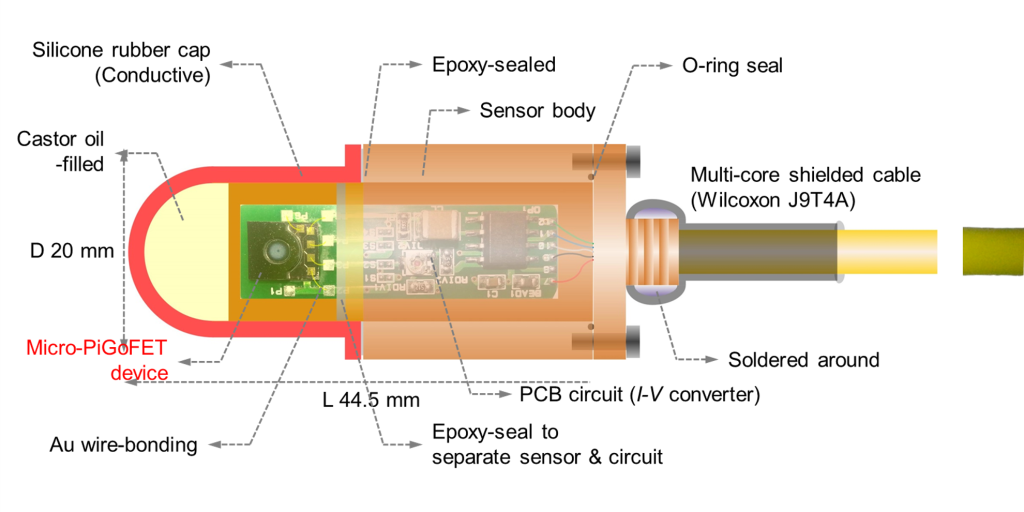
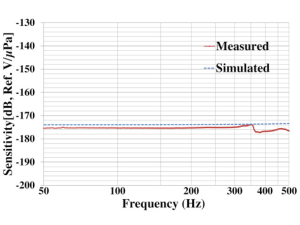
Underwater Acoustic Applications
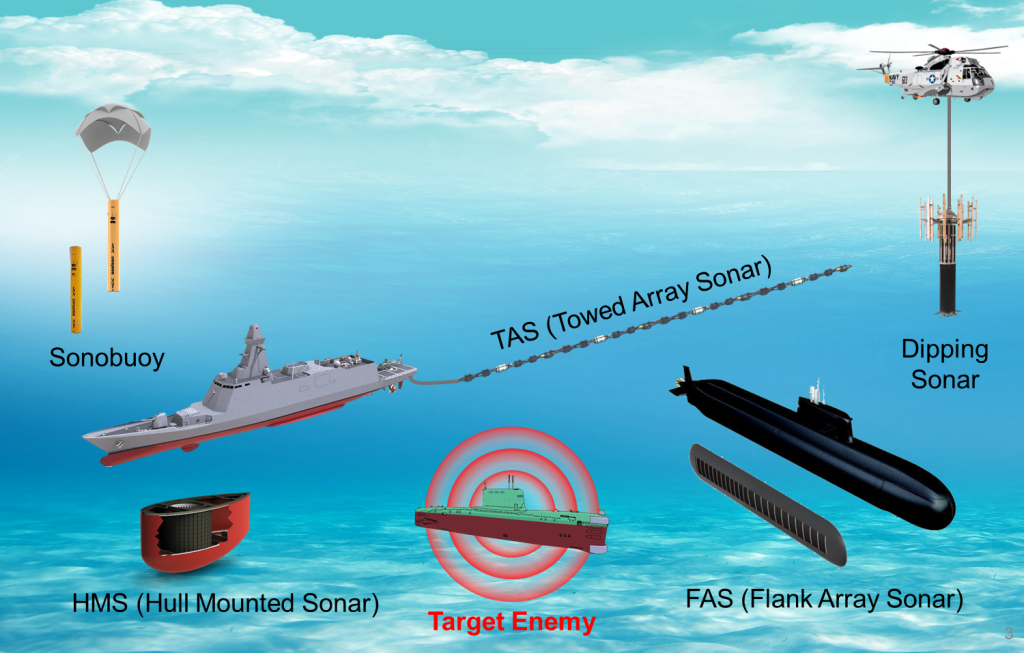
Related Publications
- Sung, M., K. Shin, and W. Moon, A micro-machined hydrophone employing a piezoelectric body combined on the gate of a field-effect transistor. Sensors and Actuators a-Physical, 2016. 237: p. 155-166.
- Sung, M., K. Shin, and W. Moon, A new transduction mechanism for hydrophones employing piezoelectricity and a field-effect transistor. Sensors and Actuators a-Physical, 2015. 233: p. 557-568.